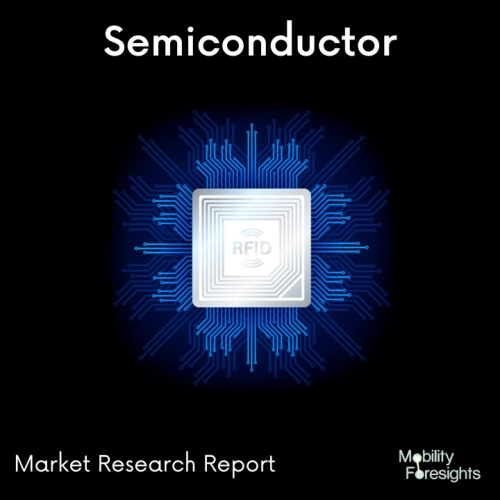
- Get in Touch with Us
Last Updated: Apr 25, 2025 | Study Period: 2024-2030
A graphene channel sandwiched between two electrodes and a gate contact that modifies the channel's electrical response makes up a graphene field effect transistor (GFET). To enable channel surface functionalization and receptor molecule binding to the channel surface, graphene is exposed. Using a field-effect transistor, the voltage on the gate of a transistor controls whether the other two terminals may conduct current or not (the source and drain).
Due to graphene's greater electrical and thermal conductivity, it dissipates heat more effectively than silicon and with lower resistance losses. As a result, graphene transistors could offer improved performance and efficiency. The channel is entirely on the surface due to the structure's one-atom thickness. The gate, which is a chip component that turns transistors on and off, is a crucial measure of transistor size. In the past, gate lengths had already been reduced to less than one nanometer. The most recent work sets a new standard that will be difficult to match by reducing gate lengths to the size of a single atom.
For high-speed analog VLSI, RF, and biosensor circuits, G-FET is emerging as the best alternative to silicon due to factors like superior carrier mobility and very high trans-conductance gain, among others. Graphene is one such material. The Junction Field-Effect Transistor (JFET) and the "Metal-Oxide Semiconductor" Field-Effect Transistor (MOSFET), also known as Insulated-Gate Field-Effect Transistor (IGFET), are the two types of field-effect transistors.
There are several advantages to using GFET sensors with a 2D channel material over bulk semiconductor devices (including silicon). The response sensitivity of the majority of semiconductor transistor sensors is limited because changes in the local electric field at the surface of the channel have little effect deeper within the device channel.
The Global Graphene Field Effect Transistors market accounted for $XX Billion in 2023 and is anticipated to reach $XX Billion by 2030, registering a CAGR of XX% from 2024 to 2030.
To minimize hurdles to graphene adoption, particularly in the market for sensors, Graphenea has started selling GFETs, or graphene field effect transistors. Researchers no longer have to stress about continuously locating high-quality GFET devices for their applications, whether in gas or biosensing or for other purposes.
GET-S10 and GET-S20 are two common GFET-for-sensing configurations that Graphenea has first released. They both have 36 individual GFETs on one square centimeter die, but they have different device layouts.The GFET-S10 has devices equally spaced over the die, whereas the GET-S20 has devices concentrated in the die's center and electrical pads near the edge.
In contrast to the GFET-S10, which contains 30 devices with the Hall bar geometry and 6 with the 2-probe geometry, the GFET-S20 devices all feature a 2-probe geometry for investigating electrical properties while sensing.
In addition to applications in graphene device development, bioelectronics, biosensing, chemical sensing, and photodetectors that the 2-probe geometry also permits, the Hall bars make magnetic field sensing possible.
Customers have the option of selecting a device layout that meets their needs. The new product is especially well suited for people who want to create unique graphene applications but lack the motivation or resources to do the extensive graphene research necessary to produce reliable, high-quality GFET devices.
Graphene makes a good sensor due to its 2D structure and superior electrical, optical, and mechanical capabilities.
The University of Manchester team's ability to detect the adsorption of a single gas molecule was the most stunning example of the power of the sensor, while several other applications in chemical, biological, or magnetic sensors as well as photodetectors were also proven.
On a GFET platform, all of those ultrasensitive devices run. The new Graphenea devices have a defined carrier mobility above 1000 cm2/V*s, residual charge carrier density under 2 x 1012cm-2, a Dirac point between 10 and 40V, and a yield over 75%.The typical Si/SiO2 substrate is used to create the GFETs, and the metal contacts are Ni/Al.
Béraud, A graphene field effect transistor (GFET) has a gate contact that modulates the channel's electronic response and consists of a graphene channel between two electrodes. By exposing the graphene, functionalization of the channel surface and receptor molecule binding to the channel surface are made possible.
The basic GFET has three terminals and shares some similarities with the conventional FET. It has a top or back gate, a drain, and a source. The GFET has a thin graphene channel between the source and drain metal electrodes, typically tens of microns thick, in contrast to a silicon-based transistor.
SL No. | Topic |
1 | Market Segmentation |
2 | Research Methodology |
3 | Executive Summary |
4 | Potential Opportunities For The Client |
5 | Average B-2-B Price Of Graphene Field Effect Transistors, By Segments |
6 | Innovations In The Graphene Field Effect Transistors Industry , By OEMs |
7 | Key Trend In Graphene Field Effect Transistors Market |
8 | Major Driver For Graphene Field Effect Transistors Market |
9 | New Development In The Graphene Field Effect Transistors Industry |
10 | New Foundries Set Up And Impact On Graphene Field Effect Transistors Market |
11 | Classification Of GFET Configurations And Gate Bias |
12 | Software Development In Graphene Field Effect Transistors In Market |
13 | Hardware Development In Graphene Field Effect Transistors In Market |
14 | Rise In Usage Of Graphene Field Effect Transistors In The Various Industry |
15 | Market Size, Dynamics And Forecast By Geography, 2024-2030 |
16 | Market Size, Dynamics And Forecast By Industry, 2024-2030 |
17 | Market Size, Dynamics And Forecast By Application, 2024-2030 |
18 | Competitive Landscape |
19 | Market Share Of Major Players-2023 |
20 | Investments & Growth Strategies Of Major Companies |
21 | M&A Activity In Past 2 Years |
22 | Company Profiles |
23 | Conclusion |