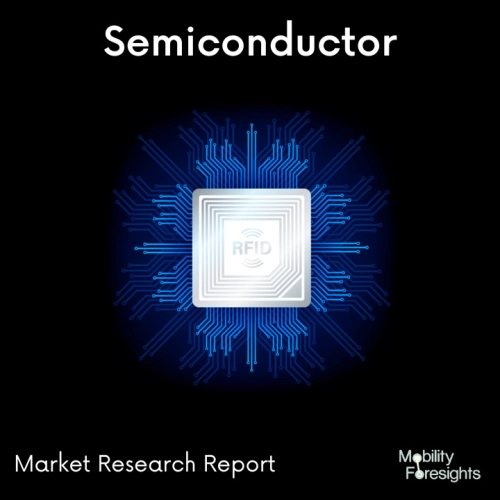
- Get in Touch with Us
Last Updated: Apr 25, 2025 | Study Period: 2023-2030
A microwave cavity sensor is a device used to measure the amount of energy present in a cavity filled with electromagnetic radiation. The cavity is typically made of metal and has walls that reflect the microwaves, creating what is known as a standing wave.
By measuring the standing wave, the sensor can detect changes in the energy within the cavity. This can be used to measure a variety of physical properties, such as temperature, pressure, or humidity.
The microwave cavity sensor works by measuring the standing wave that is created when the microwaves are reflected off of the walls. The sensor will measure the peak and trough of the standing wave, which is known as the cavity resonance frequency.
This frequency is then used to determine the amount of energy present in the cavity. The sensor can then be used to measure the physical properties of the cavity, such as temperature, pressure, or humidity.
The microwave cavity sensor is a very useful device, as it can be used to measure a variety of physical parameters in a variety of environments. This makes it an ideal choice for many applications, such as measuring the temperature in a refrigerator or monitoring the pressure within a laboratory.
The sensor can also be used for medical purposes, such as monitoring the oxygen levels in a patientâs blood. The microwave cavity sensor is a versatile device that can be used in a wide range of applications. Its ability to accurately measure physical parameters makes it a very useful tool for many industries.
The Global Microwave Cavity Sensor market accounted for $XX Billion in 2022 and is anticipated to reach $XX Billion by 2030, registering a CAGR of XX% from 2023 to 2030.
On the basis of a half-wave coaxial microwave cavity, a high-sensitivity sensor was developed for determining the amount of moisture in the air or air humidity at low pressure.
Since the cavity, or the full volume occupied by the electromagnetic field, serves as the "measuring part" of the sensor, the cavity-based methods - among the different resonant methods - offer the highest sensitivity when it comes to measuring the dielectric properties of gaseous media.
Cavity sensors' huge dimensions make them impractical for practical use at low frequencies (hundreds of MHz). Furthermore, for the same permittivity fluctuations, the absolute value of the frequency shift is smaller at low frequencies, complicating the measurement process and in the end reducing sensitivity.
A cavity sensor with a characteristic size of around 10 cm that operates in the decimeter wavelength region is a trade-off solution. Such a sensor is quite compact in size, but it also combines ease of fabrication with a reasonable level of sensitivity.
The pressure-dependent dielectric permittivity of the cavity gas determines the change in the cavity eigenfrequency. In the sixth or seventh decimal place, the value of ε deviates from unity for low gas pressures, which range from zero to several Torr. It turns out that the relative variations in the cavity eigenfrequency, which ought to be noticed, are of the same order of magnitude.
The selected design guarantees a high enough degree of mechanical stiffness and sensor parameter stability under external (mechanical, thermal, etc.) impacts. Half-wave cavity sensors are similar in size to quarter-wave sensors, but half-wave sensors have several advantages over quarter-wave sensors.
These advantages include a higher working frequency and a greater quality factor because of fewer radiation losses, which allows for an improvement in sensitivity.
They employed a number of microwave cavity prototype variations with frequencies of operation ranging from 1 to 2 GHz. The primary sensor utilised in the experimental investigations was a half-wave coaxial cavity measuring L = 95 mm in length. It was short-circuited at both ends using copper plates.
S NO | Overview of Development | Development Detailing | Region of Development | Possible Future Outcomes |
1 | A new 12m x 180° triple detection outdoor PIR + Microwave sensor with anti-masking technology has been released by TAKEX America Inc., a prominent Japanese manufacturer of intruder detection sensors using active and passive infrared technology. | MX- 12FAMD will only send an alert when detection happens over two PIR zones and microwave at the same time, using K-band microwave to identify the size and speed of an intruder. This triple detection technique can significantly reduce nuisance activations in tough situations. Dual alarm signal outputs are also featured, allowing one to select between independent outputs for each 90-degree left and right. The MX-12 AMD can blend in with any surroundings because of its single color-matched lens design. The optical heads have been simplified behind the cover into permanent but independent 90-degree left and right sides, each with individual PIR and Microwave control for optimum placement flexibility. MX-12FAMD contains their unique anti-masking technology with near-lens awareness designed to uncover efforts to obfuscate the sensor, which has now been tweaked to include four sensitivity settings to accommodate any situation. Furthermore, front and rear tampers can detect interference or attempted theft of the sensor at any time of day or night for total peace of mind protection at all times. | Global | This would enhance better innovations |
Sl no | Topic |
1 | Market Segmentation |
2 | Scope of the report |
3 | Abbreviations |
4 | Research Methodology |
5 | Executive Summary |
6 | Introdauction |
7 | Insights from Industry stakeholders |
8 | Cost breakdown of Product by sub-components and average profit margin |
9 | Disruptive innovation in theIndustry |
10 | Technology trends in the Industry |
11 | Consumer trends in the industry |
12 | Recent Production Milestones |
13 | Component Manufacturing in US, EU and China |
14 | COVID-19 impact on overall market |
15 | COVID-19 impact on Production of components |
16 | COVID-19 impact on Point of sale |
17 | Market Segmentation, Dynamics and Forecast by Geography, 2023-2030 |
18 | Market Segmentation, Dynamics and Forecast by Product Type, 2023-2030 |
19 | Market Segmentation, Dynamics and Forecast by Application, 2023-2030 |
20 | Market Segmentation, Dynamics and Forecast by End use, 2023-2030 |
21 | Product installation rate by OEM, 2023 |
22 | Incline/Decline in Average B-2-B selling price in past 5 years |
23 | Competition from substitute products |
24 | Gross margin and average profitability of suppliers |
25 | New product development in past 12 months |
26 | M&A in past 12 months |
27 | Growth strategy of leading players |
28 | Market share of vendors, 2023 |
29 | Company Profiles |
30 | Unmet needs and opportunity for new suppliers |
31 | Conclusion |
32 | Appendix |