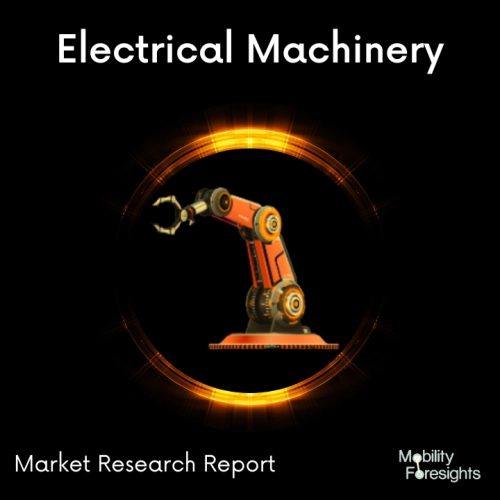
- Get in Touch with Us
Last Updated: Apr 25, 2025 | Study Period: 2024-2030
Silicon drift detectors (SDDs) are X-ray radiation detectors used in electron microscopy and x-ray spectrometry (XRF and EDS). Silicon drift detectors, like other solid state X-ray detectors, determine the energy of an incoming photon by the degree of ionisation it causes in the detector material.
The detector electronics measure the charge produced by this changing ionisation for each incoming photon. This material, high purity silicon with extremely little leakage current, is used in SDDs. Because of its great purity, Peltier cooling can be used instead of the more conventional liquid nitrogen.
The transversal field produced by a sequence of ring electrodes, which causes charge carriers to "drift" to a small collection electrode, is the primary characteristic that sets an SDD apart from other semiconductor devices.
The collection electrode, which serves as the first stage of amplification in previous detector designs, is centrally positioned with an external FET (field effect transistor) to transform the current into a voltage.
Modern designs directly include the FET into the chip, dramatically enhancing energy resolution and throughput. This is caused by a decrease in anode-to-FET capacitance, which lowers electronic noise.
Some plans relocate the anode and FET outside the radiation-exposed region. This results in a slightly slower throughput and a little longer reaction time. Yet, improved energy resolutions result from the lower anode size. Maintaining the silicon drift detector's energy resolution is attainable when combined with enhanced or modified signal processing.
The Global Silicon Drift DetectorMarket accountedfor $XX Billion in 2023 and is anticipated to reach $XX Billion by 2030, registering a CAGR of XX% from 2024 to 2030.
A negative bias voltage is applied to both sides of a thick disc of high-resistance silicon to cause it to completely deplete. This is how Silicon Drift Detectors (SDD) work. A significant transverse electric field component is created within the structure on one side of the planar structure where the bias is graduated throughout the device using a series of "drift rings."
This is done to send electrons created by x-ray interactions in the direction of a tiny anode that collects charge. To enable good low energy x-ray sensitivity and minimal charge leakage, the device has a consistent shallow implanted junction contact on the other side.
The very low capacitance of this drift detector construction allows for good energy resolution at reasonably fast electronic processing times.
As Silicon Drift Detectors have a very low leakage current, they can operate at temperatures that electronic Peltier cooling systems can easily attain, negating the need for liquid nitrogen and enabling maximum performance. A low noise charge sensitive amplifier and an input FET (field effect transistor) that is localised at the sensor make up the preamplifier.
Signal electrons are transmitted to the FET gate after being captured at the anode by the drift field in the sensor. Via the use of a feedback capacitor Cf, the preamplifier converts this signal charge Qs into a voltage step Vstep.
SDDs are a promising technology with a lot of potential for growth. The key players in the market are well-positioned to benefit from this growth by investing in research and development, expanding their manufacturing capacity, and launching new products that meet the needs of the growing market.
Bruker Corporation, a leading provider of analytical instruments, has launched a new silicon drift detector (SDD) for electron microscopy. The new SDD, called the XFlash 7, offers improved energy resolution and count rates compared to previous models. This makes it ideal for a wide range of electron microscopy applications, such as materials science, biology, and semiconductors.
Hitachi High-Technologies Corporation, a Japanese multinational electronics company, has launched a new silicon drift detector (SDD) for X-ray fluorescence (XRF) analysis. The new SDD, called the EX-500, offers improved energy resolution and count rates compared to previous models. This makes it ideal for a wide range of XRF analysis applications, such as elemental analysis, alloy analysis, and quality control.
Oxford Instruments plc, a British scientific instrumentation company, has launched a new silicon drift detector (SDD) for medical imaging. The new SDD, called the X-act 5, offers improved energy resolution and count rates compared to previous models. This makes it ideal for a wide range of medical imaging applications, such as X-ray computed tomography (CT) scanning and positron emission tomography (PET) scanning.
New SDD technologies are being developed to improve their energy resolution, count rates, and other performance characteristics. For example, new SDD designs with larger areas and higher count rates are being developed for industrial applications. New SDDs with improved energy resolution and low-energy response are being developed for medical applications.
The adoption of SDDs in emerging markets is increasing rapidly, driven by the increasing demand for high-performance analytical instruments in these markets. This trend is expected to continue in the coming years, as emerging markets become increasingly industrialized and urbanized.
New SDD technologies are being developed to improve their performance and expand their range of applications. For example, new SDD designs with larger areas and higher count rates are being developed for industrial applications. New SDDs with improved energy resolution and low-energy response are being developed for medical applications.
The future outlook for the global SDD market is very positive. The market is expected to grow rapidly in the coming years, driven by the increasing demand for high-resolution and high-performance X-ray detectors in a wide range of applications. The key players in the market are well-positioned to benefit from this growth by investing in research and development, expanding their manufacturing capacity, and launching new products that meet the needs of the growing market.
The EERE program is a program that provides funding for research and development of new energy technologies. The EERE program has funded a number of projects to develop new SDD technologies for solar energy applications
The MDD is a directive that sets forth the requirements for medical devices that are sold in the European Union. The MDD has strict requirements for the safety and efficacy of medical devices. These requirements have helped to drive the adoption of SDDs in medical imaging applications in the EU.
The Made in China 2025 initiative is a government initiative to upgrade China's manufacturing sector. The initiative has set a goal of China becoming a leading manufacturer of semiconductors and other high-tech products. This initiative is expected to drive the demand for SDDs in China, as SDDs are used in a variety of high-tech manufacturing applications.
Bruker Corporation, a leading provider of analytical instruments, has launched a new silicon drift detector (SDD) for electron microscopy. The new SDD, called the XFlash 7, offers improved energy resolution and count rates compared to previous models. This makes it ideal for a wide range of electron microscopy applications, such as materials science, biology, and semiconductors.
Hitachi High-Technologies Corporation, a Japanese multinational electronics company, has launched a new silicon drift detector (SDD) for X-ray fluorescence (XRF) analysis. The new SDD, called the EX-500, offers improved energy resolution and count rates compared to previous models. This makes it ideal for a wide range of XRF analysis applications, such as elemental analysis, alloy analysis, and quality control.
Oxford Instruments plc, a British scientific instrumentation company, has launched a new silicon drift detector (SDD) for medical imaging. The new SDD, called the X-act 5, offers improved energy resolution and count rates compared to previous models. This makes it ideal for a wide range of medical imaging applications, such as X-ray computed tomography (CT) scanning and positron emission tomography (PET) scanning.
A number of projects are underway to develop next-generation SDDs with even higher energy resolution, count rates, and other performance characteristics. These new SDDs are expected to enable new applications and improve the performance of existing applications.
The European Union's Horizon 2020 program is funding a number of projects to develop new SDD technologies and applications. For example, the EU is funding a project called "SDD4Industry" to develop SDDs for industrial applications. The EU is also funding a project called "SDD4Medical" to develop SDDs for medical applications.
The US government's Department of Energy is funding a number of projects to develop SDDs for solar energy applications. For example, the DOE is funding a project to develop SDDs for X-ray fluorescence (XRF) analysis of solar cells.
A number of companies are also developing new SDD technologies and applications. For example, Bruker Corporation is developing new SDDs for electron microscopy and XRF analysis. Oxford Instruments is developing new SDDs for medical imaging.
Sl no | Topic |
1 | Market Segmentation |
2 | Scope of the report |
3 | Abbreviations |
4 | Research Methodology |
5 | Executive Summary |
6 | Introduction |
7 | Insights from Industry stakeholders |
8 | Cost breakdown of Product by sub-components and average profit margin |
9 | Disruptive innovation in the Industry |
10 | Technology trends in the Industry |
11 | Consumer trends in the industry |
12 | Recent Production Milestones |
13 | Component Manufacturing in US, EU and China |
14 | COVID-19 impact on overall market |
15 | COVID-19 impact on Production of components |
16 | COVID-19 impact on Point of sale |
17 | Market Segmentation, Dynamics and Forecast by Geography, 2024-2030 |
18 | Market Segmentation, Dynamics and Forecast by Product Type, 2024-2030 |
19 | Market Segmentation, Dynamics and Forecast by Application, 2024-2030 |
20 | Market Segmentation, Dynamics and Forecast by End use, 2024-2030 |
21 | Product installation rate by OEM, 2023 |
22 | Incline/Decline in Average B-2-B selling price in past 5 years |
23 | Competition from substitute products |
24 | Gross margin and average profitability of suppliers |
25 | New product development in past 12 months |
26 | M&A in past 12 months |
27 | Growth strategy of leading players |
28 | Market share of vendors, 2023 |
29 | Company Profiles |
30 | Unmet needs and opportunity for new suppliers |
31 | Conclusion |
32 | Appendix |