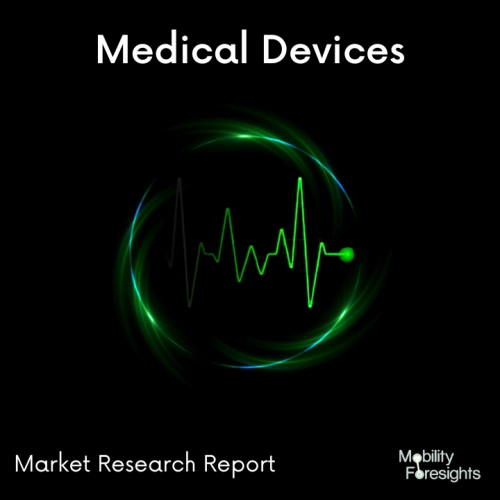
- Get in Touch with Us
Last Updated: Apr 25, 2025 | Study Period: 2024-2030
The Global Two-Photon Laser Scanning Confocal Microscope Market is a niche but growing sector in the world of scientific instrumentation. This advanced imaging technology offers researchers and scientists the ability to explore biological and material samples with unprecedented detail and precision.
The Two-Photon Laser Scanning Confocal Microscope is a specialized imaging tool used primarily in scientific research, particularly in the fields of biology, material science, and neuroscience. It employs cutting-edge laser technology to produce high-resolution, three-dimensional images of samples. The Two-Photon Laser Scanning Confocal Microscope market is steadily growing. The market size is relatively small but has been expanding due to the increasing demand for high-quality imaging and the advancement of research in various fields.
This microscopy technology finds applications in various scientific domains. It is widely used for imaging live cells, deep tissue imaging, brain mapping, and investigating the structural and functional properties of materials. Neuroscientists, biologists, and materials scientists are among the primary users of this technology.
The market is driven by continuous technological advancements in laser sources, detectors, and imaging software. These improvements have led to enhanced imaging capabilities, including deeper penetration into samples and increased resolution.
Key benefits of two-photon microscopy include the ability to visualize living tissue at substantial depths, a feat unattainable with one-photon techniques. Its primary functions involve optical sectioning for 3D reconstructions and generating a point light source while excluding out-of-focus light, thus facilitating deep-tissue imaging with exceptional clarity.
Notably, two-photon excitation microscopy utilizes near-infrared (NIR) light, which reduces tissue scattering and background noise. This, along with its superior tissue penetration and reduced photobleaching, positions it as a promising alternative to traditional confocal microscopy.
In practice, two-photon microscopy shares similarities with other laser microscopy methods, such as Raman and laser scanning confocal microscopy. These techniques employ optical sectioning and laser beams focused in a raster pattern to form images.
Unlike confocal microscopes, multiphoton microscopes lack pinhole apertures for optical sectioning. Instead, they leverage the unique point spread function of excitation, which enhances optical sectioning.
The underlying theory of two-photon excitation involves the simultaneous excitation of a fluorophore by two lower-energy photons, each carrying about half the required excitation energy. This results in the emission of a fluorescence photon with higher energy and a shorter wavelength.
The key advantage is the minimal likelihood of simultaneous absorption of two photons, which necessitates the use of femtosecond pulsed lasers to create a high peak flux of excitation photons. This unique effect significantly reduces the axial spread of the point spread function, improving imaging along the z-axis and allowing for precise adjustments in spot size and shape for specific objectives.
Moreover, the longer wavelength and lower energy of the excitation lasers, often in the infrared range, make two-photon microscopy ideal for imaging live cells with minimal damage. This capability enables longer observation periods with fewer adverse effects.
The Global Two-Photon Laser Scanning Confocal Microscope market accounted for $XX Billion in 2023 and is anticipated to reach $XX Billion by 2030, registering a CAGR of XX% from 2024 to 2030.
Precision fluorescence microscopy has been improved upon by two-photon microscopy, also known as two-photon laser scanning microscopy.A single photon of light with a certain wavelength activates a fluorophore to emit light in confocal or epifluorescent microscopy. A fluorophore can be stimulated in two-photon microscopy by simultaneously absorbing two photons. Each photon in this scenario has a wavelength that is twice that of the typical excitation wavelength, giving each photon half its normal energy level.
When two lower-energy photons strike a fluorophore at the same moment, they each contribute half of the excitation energy required to interact with the fluorophore as if only one photon with a higher energy and shorter wavelength. The key benefit of this approach is that fluorescence activation is limited to a small plane of focus inside the specimen because the near-simultaneous arrival of two photons is an incredibly unusual event.
Even when there are only a few fluorophores present, this offers crisper images than conventional confocal microscopy because photons are only released from excited fluorophores that are present at the focal plane. This enables the collection of all emitted light. Background fluorescence cannot be produced because there is not enough energy to activate fluorophores above and below the focal plane.
Compared to confocal or epifluorescence microscopy, two-photon microscopy may reach deeper into tissue since the excitation wavelength is roughly twice as long.Longer wavelength light scatters less, allowing deeper tissue penetration. With little disruption to the underlying physiology, two-photon microscopy offers a perfect platform for imaging dynamic biological processes in deep tissue of living animals.
Without specific laser physics training, biologists may now operate two-photon microscopes thanks to recent advancements in ultrafast laser technology. Thus, clear recommendations are required to encourage the adoption and use of two-photon microscopy.
Two-photon microscopy is particularly helpful for extended viewing of cells in undissected, living brain tissue in entire animals or fine-scale neuronal structures in brain slices because excitation is constrained to a focused point and results in reduced photobleaching and phototoxicity. The main drawback of this approach is the high cost and specialized equipment needed to set up a two-photon microscope.
Multiphoton microscopy, also known as two-photon excitation microscopy (2PEM), was developed approximately three decades ago and represents a fluorescent imaging technique that enables the observation of cells at depths beyond the capability of confocal microscopy.
In this method, when appropriate light irradiates fluorophores, the absorbed light triggers electron transfer to an excited state. Subsequently, energy is emitted as a photon as electrons return to their ground state, resulting in fluorescence emission. This process involves a linear single-photon absorption when one photon excites a fluorophore.
In two-photon absorption, a fluorophore simultaneously absorbs two photons, each approximately double the wavelength. To achieve such a rare two-photon absorption, 2PEM employs femtosecond pulsed lasers, producing an exceptionally high photon density.
2PEM leverages nonlinear optical phenomena, which respond non-linearly to the intensity of the applied optical field, owing to the intense light generated by lasers. The multiphoton absorption is spatially confined to the perifocal region under nonlinear contrast mechanisms of photon density. This results in increased tissue viability and reduced overall photodamage, except in the immediate vicinity of the focal point.
Moreover, 2PEM commonly employs near-infrared (NIR) wavelengths for sample stimulation, allowing deep penetration into biological structures. NIR light is less phototoxic and penetrates deeper tissues while experiencing reduced endogenous absorption by most tissues. As a result, 2PEM has found extensive use in biological research, encompassing neuroscience at considerable depths, in vivo animal pathology, and plant studies. It has emerged as an indispensable tool for live-tissue investigations.
In addition to live-cell imaging, there has been recent progress in deep two-photon imaging of fixed samples through novel clearing techniques. Two-photon lasers, frequently utilizing femtosecond Ti:sapphire sources with tunable excitation wavelengths, have enabled the observation of dynamic subcellular events.
To enhance the optical resolution and micrograph contrast, confocal laser scanning microscopy (CLSM) employs a spatial pinhole to reject out-of-focus fluorescent light. While CLSM is a potent tool for biological imaging in plant studies, it presents certain limitations.
Visible-light lasers used in confocal lasers cause heat and light to scatter outside the focal plane, potentially leading to photodamage and photobleaching in biological tissues. Additionally, the high light dispersion and plant-specific autofluorescence can hinder fluorescent signal detection in deep tissue imaging, making CLSM less effective in this regard.
Sl no | Topic |
1 | Market Segmentation |
2 | Scope of the report |
3 | Research Methodology |
4 | Executive Summary |
5 | Average B2b Price, By Region |
6 | Potential Opportunities For Client |
7 | Introduction |
8 | Insights from Industry stakeholders |
9 | Disruptive innovation in the Industry |
10 | Technology trends in the Industry |
11 | Consumer trends in the industry |
12 | Recent Production Milestones |
13 | Key Drivers Of Global Two-Photon Laser Scanning Confocal Microscope market |
14 | Advances in Laser Technologies for Two-Photon Microscopy |
16 | New product development in past 12 months |
17 | Market Segmentation, Dynamics and Forecast by Geography, 2024-2030 |
18 | Market Segmentation, Dynamics and Forecast by Product Type, 2024-2030 |
19 | Market Segmentation, Dynamics and Forecast by Application, 2024-2030 |
20 | Market Segmentation, Dynamics and Forecast by End use, 2024-2030 |
21 | Competitive Landscape |
22 | M&A in past 12 months |
23 | Growth strategy of leading players |
24 | Market share of vendors, 2023 |
25 | Company Profiles |
26 | Unmet needs and opportunity for new suppliers |
27 | Conclusion |