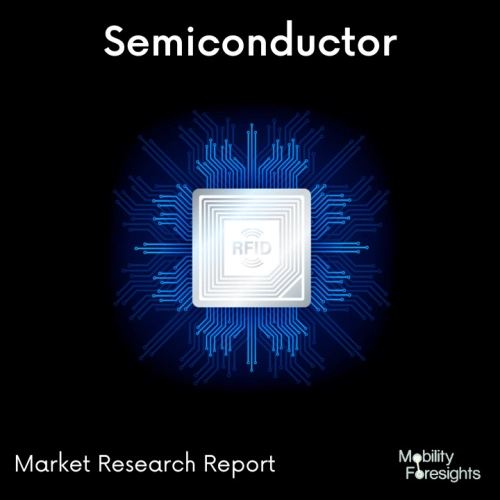
- Get in Touch with Us
Last Updated: Apr 25, 2025 | Study Period: 2023-2030
Research in a variety of fields uses the chemical state analysis method known as X-ray absorption spectroscopy (XAS). By measuring the transmission (or fluorescence) of x-rays as a function of increasing x-ray energy incrementally in small steps at energies near to the absorption edge, this method can be used to study biological tissues.
The energy needed to eject an electron from an electron shell) of an element of interest (such as Fe) is the same as the absorption edge energy. The status of the electrons can be inferred from subtle alterations in the way x-rays are absorbed close to an atom's absorption edge.
There are two regions in X-ray Absorption Spectroscopy (XAS):Sharp resonance peaks can be seen in the region of the x-ray spectrum closest to the absorption edge (about 100 eV about the edge), which is known as the X-ray absorption near edge structure (XANES/NEXAFS).
In general, the area is sensitive to local atomic states like symmetry and oxidation states.Extended fine structure (EXAFS): Features in this region can be found up to 1000 eV or more beyond the absorption edge, emerging after the XANES region.
EXAFS is characterized by mild oscillations in the recorded signal and is brought on by the scattering of the expelled electron by the atoms in the immediate vicinity. The information about nearby atoms that can be measured via EXAFS includes bond lengths and chemical coordination environments.
The Global X-ray Absorption Spectroscopy (XAS) market accounted for $XX Billion in 2022 and is anticipated to reach $XX Billion by 2030, registering a CAGR of XX% from 2023 to 2030.
Standard experimental station for effective X-ray Absorption Spectroscopy (XAS): Hutch BFor effective X-ray Absorption Spectroscopy (XAS) on samples put into conventional sample holders, the first experimental hutch is designed.
This hutch houses a non-customizable optical table with enough equipment to reduce beamline downtime. On samples tested in a cryostat (at 10 K) or a chamber with a constant temperature of room air, we support transmission and fluorescence.
Additionally, if the cell fits inside the room temperature chamber's space envelope, the X-Y stages operating at room temperature can handle small user-supplied cells (such as those used for in-situ catalysis).
Similar to that, ANSTO also supports capillary heating to 1000K in Hutch B on the X-Y stage and in-situ battery study. Please get in touch with the beamline team to go over particular requirements.
An key technique for comprehending the surface chemistry of materials is X-ray photoelectron spectroscopy (XPS), which may offer information on the local bonding environment of a specific species.
As a result, the oxidation states of silicon at the surface are easily revealed by the Si 2p level examination of an oxidised silicon wafer by XPS. It has also been shown to be a special tool for describing the chemical makeup of nanoparticles' surfaces and bulks. However, anytime samples are put on a substrate, data obtained from nano-objects frequently experience interpretation issues.
New experiments based on free nanoparticles interacting with photons are being considered at the moment. A novel experimental technique to XPS is shown here, which combines soft X-ray synchrotron radiation with an in-vacuum beam of isolated nanoparticles focussed by an aerodynamic lens system.
The structure of the Si/SiO2 interface is investigated for silicon nanocrystals (Si-NCs) previously oxidised in ambient air as a proof of concept.
Under high vacuum circumstances, a beam of isolated nanoparticles crosses synchrotron radiation, allowing the interaction between a particle and the soft X-rays to be highly defined both spatially and temporally. Atomization of a nanoparticle solution results in a diphasic flow consisting of an inert carrier gas and the sample in aerosol form.
A commercial scanning mobility particle sizer (SMPS) is used to quantify the nanoaerosol mobility diameter and number density online. Under vacuum, an aerodynamic lens system (ADLS) generates a focused, continuously renewable nanoparticle beam from an aerosol stream at atmospheric pressure.
This is accomplished by passing the nano aerosol flow via a 200 m limiting entry hole, which is followed by four compartments separated by diaphragms.
Laser pyrolysis was used to initially create the Si-NCs sample. For the tests, a dry powder of Si-NCs weighing around 500 mg was synthesised.
After being synthesised, the sample was allowed to oxidise for two months in ambient air before being dissolved in ethanol and agitated ultrasonically soon before the trials. High resolution transmission electron microscopy was used to characterise it. Including the thin amorphous oxide layer that developed at the surface of the particles during air exposure.
Fluid dynamics models reveal that the ADLS can concentrate high-density spherical nanoparticles as small as 40 nm in diameter.Brownian motion has a considerable influence on smaller particle trajectories in the ADLS and at its immediate exit, resulting in a divergent beam with too low particle density for spectroscopic research employing synchrotron light.
However, one efficient technique to circumvent this challenge is to intentionally agglomerate the nanoparticles in the beam while maintaining separate primary particles.
This may be accomplished by adjusting the concentration of Si-NC in the atomized solution.The mass concentration was fixed to 1g/l in ethanol for this purpose.
Following atomization, the size of the nano aerosols was stabilised throughout time and carefully observed to have a mobility diameter of Dm = 150 nm, regardless of the duration of the ultrasonic treatment.
This mobility diameter is caused by diffusion-limited agglomeration of primary Si-NCs that takes place at the atomization stage of the suspension and in the streamline up to the ADLS outlet, and it is compatible with the ADLS focusing capabilities. It translates to 154 main particles on average per agglomeration.
Sl no | Topic |
1 | Market Segmentation |
2 | Scope of the report |
3 | Abbreviations |
4 | Research Methodology |
5 | Executive Summary |
6 | Introduction |
7 | Insights from Industry stakeholders |
8 | Cost breakdown of Product by sub-components and average profit margin |
9 | Disruptive innovation in the Industry |
10 | Technology trends in the Industry |
11 | Consumer trends in the industry |
12 | Recent Production Milestones |
13 | Component Manufacturing in US, EU and China |
14 | COVID-19 impact on overall market |
15 | COVID-19 impact on Production of components |
16 | COVID-19 impact on Point of sale |
17 | Market Segmentation, Dynamics and Forecast by Geography, 2023-2030 |
18 | Market Segmentation, Dynamics and Forecast by Product Type, 2023-2030 |
19 | Market Segmentation, Dynamics and Forecast by Application, 2023-2030 |
20 | Market Segmentation, Dynamics and Forecast by End use, 2023-2030 |
21 | Product installation rate by OEM, 2023 |
22 | Incline/Decline in Average B-2-B selling price in past 5 years |
23 | Competition from substitute products |
24 | Gross margin and average profitability of suppliers |
25 | New product development in past 12 months |
26 | M&A in past 12 months |
27 | Growth strategy of leading players |
28 | Market share of vendors, 2023 |
29 | Company Profiles |
30 | Unmet needs and opportunity for new suppliers |
31 | Conclusion |
32 | Appendix |