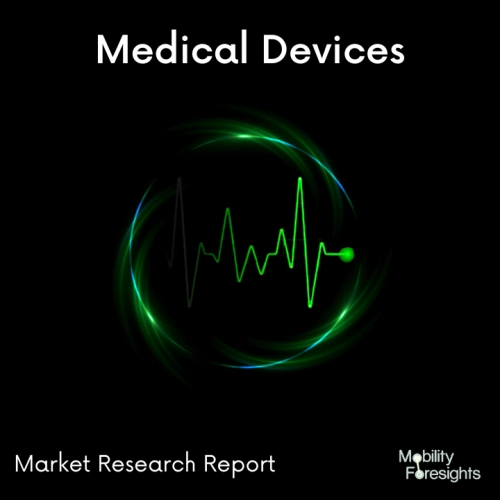
- Get in Touch with Us
Last Updated: Apr 26, 2025 | Study Period: 2023-2030
PET employs radioactive tracers to provide functional imaging of various metabolic processes, blood flow measures, regional chemical composition, and/or chemical absorption.
Different tracers are utilized for various medical problems, such as cancer, certain brain pathologies, cardiac events, and bone lesions, depending on the targeted processes within the live body, with radiolabeled tracers being the most widely employed.
The oxygen-15 isotope is typically used in blood flow measurements, although a wide range of 11C-based drugs have also been produced for neurological diseases, prostate cancer, and lung carcinomas.
The single-photon emission computed tomography (SPECT) approach, on the other hand, employs gamma-emitting radioisotopes and may be used to identify strokes, seizures, bone disorders, and infections by measuring blood flow and radioactivity.
Over the last decade, the original emphasis on medical imaging centered on detection and diagnosis has shifted to prognosis, tissue characterisation, and therapy efficacy prediction.
Functional imaging, such as positron emission tomography (PET) and single-photon emission computed tomography (SPECT), has therefore become critical in clinical decision-making in a variety of sectors of medicine.
Furthermore, hybrid imaging, which combines SPECT and PET with computed tomography (CT) or magnetic resonance imaging (MRI), has improved the diagnostic accuracy of both PET and SPECT by taking advantage of morphological information obtained from CT and MRI scans and implementing attenuation correction.
PET is a quantitative imaging technology that looks to outperform the SPECT approach. However, the solution to the contentious question of which modality will monopolize nuclear imaging technology.
When compared to SPECT, PET technology traditionally gives better picture resolution, less attenuation (due to higher photon energy), fewer scatter artifacts, and hence superior diagnostic capabilities.
PET's better sensitivity and more durable and adaptable tracers are two of the most significant benefits of PET over the SPECT modality, making PET a versatile and powerful tool for clinical and research purposes.
These benefits, however, come at a considerable cost, limiting the availability of PET imaging. Because vehicle molecules are commonly responsible for chemical and biological processes within the body, chemical linkers are used to stabilize the links between vehicle structures and radionuclides.
The ligands must be very selective and specific to their targets. These targets might include transporters, enzymes, specific receptors, or antigens.
Furthermore, the targets may be involved in metabolic changes, tissue hypo-oxygenation, or changes in gene and/or protein expression.
However, under pathological settings, the target's function may be greatly changed, changing the biological interactions between the vehicle portion and its target, especially in tumors, where the expression pathways of receptors, transporters, and enzymes are heavily modified.
Radioisotopes have short half-lives, they must be produced in-house using cyclotrons. That is the primary benefit of SPECT. Radiopharmaceuticals used in SPECT imaging are less expensive and easier to distribute, and they provide more exact targeting in some circumstances.
Because vehicle molecules are commonly responsible for chemical and biological processes within the body, chemical linkers are used to stabilize the links between vehicle structures and radionuclides.
The ligands must be very selective and specific to their targets. These targets might include transporters, enzymes, specific receptors, or antigens.
Furthermore, the targets may be involved in metabolic changes, tissue hypo-oxygenation, or changes in gene and/or protein expression.
However, under pathological settings, the target's function may be greatly changed, changing the biological interactions between the vehicle portion and its target, especially in tumors, where the expression pathways of receptors, transporters, and enzymes are heavily modified. The radiolabeled chemical preparation should be tested in an aqueous solution with a pH as near to that of blood as practicable.
Furthermore, the ionic strength and osmolality must be compatible with blood. Their sizes, weights, charges, morphologies, and a basic physicochemical feature, lipophilicity, all impact their solubility.
Last but not least, lipophilicity has a considerable influence on drug molecule absorption, distribution, and excretion.Thus, features affecting physicochemical, radiochemical, or biological qualities are also necessary through quality control testing.
The Global Molecular Imaging Agents (Radiopharmaceuticals) Market accounted for $XX Billion in 2022 and is anticipated to reach $XX Billion by 2030, registering a CAGR of XX% from 2023 to 2030.
Peptide-Based Radiopharmaceuticals for Prostate Cancer: New Frontiers in Molecular Imaging. The increasing prevalence of prostate cancer (PCa) necessitates advancements in its detection, staging, and precise therapy.
Overexpression of tumor-specific peptide receptors, such as gastrin-releasing peptide receptor, natriuretic peptide receptor, and somatostatin receptor, in human cancer cells has shown the optimal biological basis for targeted imaging and therapy.
Targeting these receptors using radiolabeled peptides and analogues is a critical area at the forefront of PCa research right now.Radiolabeled peptides have been employed with great affinity and specificity to target receptors for molecular imaging in human PCa.
The radiolabeled peptides allow for rapid clearance from blood and normal tissues, resulting in strong contrast for positron emission computed tomography and single-photon emission imaging.
Because of their efficacy in visualization, peptide derivatives with therapeutic radionuclides have been investigated in recent years for peptide receptor radionuclide treatment in PCa.
These advancements hold out the possibility of personalized, molecular treatment for specific patients. As a result, evaluate the preclinical and clinical literature from the previous years and concentrate on the most recent breakthroughs in peptide-based radiopharmaceuticals for PCa imaging and treatment.
Smart MR Imaging Agents Relevant to Potential Neurologic Applications. Molecular imaging is a new field that seeks to noninvasively visualise the expression and activity of bioactive molecules, which frequently indicate particular molecular markers in disease processes.
It investigates the underlying biochemical or physiologic abnormalities of the disease rather than the structural manifestations of these abnormalities. Although the potential therapeutic uses of molecular imaging have been widely recognised, the existing molecular imaging techniques' poor sensitivity and specificity are frequently a major hurdle for clinical applications. Aside from clinical situations, the use of molecular imaging can greatly help drug development.
Molecular imaging provides reproducible real-time visualisation of biologically relevant processes, which can replace common invasive research techniques like histology, which necessitates animal sacrifice at each time point in the experiment.
It also enables rationalisation of parameters related to disease processes. At the moment, three degrees of simultaneous advancement are being made in the field of molecular imaging: 1) enhancing imaging hardware for use in preclinical and clinical contexts; 2) finding and confirming novel physiologically relevant imaging targets; and 3) creating inventive imaging probes.
Because molecular imaging, in contrast to anatomic imaging, always necessitates the use of a molecular imaging probe, chemical development assumes a vital role.Magnetic resonance imaging is the most appealing of all the molecular imaging modalities. Because of its outstanding resolution, it may be used to map biomarker activity while also obtaining anatomical pictures.
Contrast-enhancing drugs have been utilised extensively since MR imaging was first employed in clinical settings to raise the comparatively poor sensitivity of the method. The paramagnetic materials used as contrast agents in conventional MR imaging increase the rates of water proton relaxation.
In contrast to optical or radiodiagnostic probes, MR imaging agents are identified by their impact on the relaxation of the water proton rather than by directly producing a signal intensity.
Nowadays, most applications of Gd3+ in clinical practice are related to its poly(amino carboxylate) complexes due to its strong paramagnetism (it contains seven unpaired electrons).
When it comes to longitudinal relaxation periods (T1 agents) as opposed to transverse relaxation times (T2 agents), Gd3+ complexes have a greater impact. To stop the Gd3+ ion from becoming harmful, a poly(amino carboxylate) ligand, such DTPA or DOTA, wraps around it in the Gd3+ complexes. This allows space for one water molecule to be directly connected to the paramagnetic metal ion.
This water molecule's interchange with the surrounding water is crucial because it will allow the Gd's paramagnetic action to be transmitted to the bulk water, allowing the molecule to be detected as a shortened proton relaxation period.Clinical agents that are currently in use lack specificity.
Although they are useful in defining the structural anatomical effects of illnesses, they are unable to identify the underlying biochemical or physiological problems. To develop a "smart" (activatable or responsive) contrast agent, the agent's relaxivityâthe ability to accelerate water proton relaxationâmust be selectively altered by the specific parameter or biomarker that needs to be detected.
Sl no | Topic |
1 | Market Segmentation |
2 | Scope of the report |
3 | Abbreviations |
4 | Research Methodology |
5 | Executive Summary |
6 | Introduction |
7 | Insights from Industry stakeholders |
8 | Cost breakdown of Product by sub-components and average profit margin |
9 | Disruptive innovation in the Industry |
10 | Technology trends in the Industry |
11 | Consumer trends in the industry |
12 | Recent Production Milestones |
13 | Component Manufacturing in US, EU and China |
14 | COVID-19 impact on overall market |
15 | COVID-19 impact on Production of components |
16 | COVID-19 impact on Point of sale |
17 | Market Segmentation, Dynamics and Forecast by Geography, 2023-2030 |
18 | Market Segmentation, Dynamics and Forecast by Product Type, 2023-2030 |
19 | Market Segmentation, Dynamics and Forecast by Application, 2023-2030 |
20 | Market Segmentation, Dynamics and Forecast by End use, 2023-2030 |
21 | Product installation rate by OEM, 2023 |
22 | Incline/Decline in Average B-2-B selling price in past 5 years |
23 | Competition from substitute products |
24 | Gross margin and average profitability of suppliers |
25 | New product development in past 12 months |
26 | M&A in past 12 months |
27 | Growth strategy of leading players |
28 | Market share of vendors, 2023 |
29 | Company Profiles |
30 | Unmet needs and opportunity for new suppliers |
31 | Conclusion |
32 | Appendix |