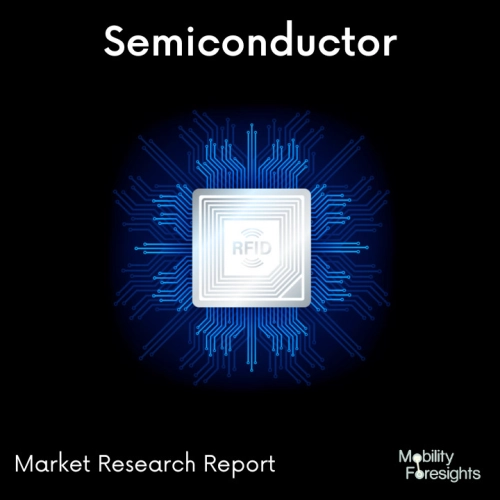
- Get in Touch with Us
Last Updated: Apr 25, 2025 | Study Period: 2024-2030
An essential method for determining the binding affinity of ligands and proteins is fluorescence quenching. Fluorescence quenching, which is caused by a number of chemical interactions with a quencher molecule, is the reduction in the quantum yield of fluorescence from a fluorophore (s).
Any procedure that lessens the intensity of a sample's fluorescence is referred to as fluorescence quenching. Quenching can happen as a result of numerous molecular interactions. They include molecular rearrangements, energy exchange, the production of ground-state complexes, and collisional quenching.
The data analysis may be complicated by static quenching as well. In addition to the previously mentioned mechanisms, apparent quenching can also happen because of the sample's optical characteristics. Fluorescence intensity can be lowered by high optical density or turbidity.
Many studies have been conducted on fluorescence quenching, both as a fundamental phenomenon and as a source of knowledge about biological systems.
The molecular interactions that cause quenching are what lead to these biological applications of quenching. The fluorophore and quencher must make molecular contact for both static and dynamic quenching to occur.
The quencher must diffuse to the fluorophore during the duration of the excited state in the case of collisional quenching. The fluorophore returns to the ground state upon contact, emitting no photons. In most cases, quenching takes place without the molecules changing permanently, i.e. without a photochemical reaction.
A non fluorescent compound between the fluorophore and the quencher is generated during static quenching. The fluorophore and quencher must be in contact for either static or dynamic quenching to take place.
The Global quenched fluorescence Analyzer market accountedfor $XX Billion in 2023 and is anticipated to reach $XX Billion by 2030, registering a CAGR of XX% from 2024 to 2030.
The many uses of quenching are a result of the necessity of molecule interaction for quenching. For instance, quenching measurements can show how easily quenchers can access fluorophores. Think about a fluorophore that is attached to a membrane or a protein.
Both collisional and static quenching are impossible if the protein or membrane is impermeable to the quencher and the fluorophore is inside the macromolecule.
Because of this, quenching studies can be used to determine where fluorophores are located in proteins and membranes as well as how permeable they are to quenchers. The diffusion coefficient of the quencher can also be calculated using the rate of collisional quenching.
Any procedure that lessens a substance's fluorescence intensity is referred to as quenching. Many different processes, including excited state reactions, energy transfer, complex-formation, and collisional quenching, can cause quenching.
As a result, pressure and temperature play a big role in quenching. Common chemical quenchers include iodide ions, acrylamide, and molecular oxygen. The well-known quencher for quinine fluorescence is the chloride ion.
For non-instant spectroscopic techniques like laser-induced fluorescence, quenching presents a challenge. Optode sensors utilise quenching; for example, the quenching effect of oxygen on specific ruthenium complexes enables the detection of oxygen saturation in solution. Forster resonance energy transfer (FRET) tests are based on quenching.
Sl no | Topic |
1 | Market Segmentation |
2 | Scope of the report |
3 | Abbreviations |
4 | Research Methodology |
5 | Executive Summary |
6 | Introduction |
7 | Insights from Industry stakeholders |
8 | Cost breakdown of Product by sub-components and average profit margin |
9 | Disruptive innovation in the Industry |
10 | Technology trends in the Industry |
11 | Consumer trends in the industry |
12 | Recent Production Milestones |
13 | Component Manufacturing in US, EU and China |
14 | COVID-19 impact on overall market |
15 | COVID-19 impact on Production of components |
16 | COVID-19 impact on Point of sale |
17 | Market Segmentation, Dynamics and Forecast by Geography, 2024-2030 |
18 | Market Segmentation, Dynamics and Forecast by Product Type, 2024-2030 |
19 | Market Segmentation, Dynamics and Forecast by Application, 2024-2030 |
20 | Market Segmentation, Dynamics and Forecast by End use, 2024-2030 |
21 | Product installation rate by OEM, 2023 |
22 | Incline/Decline in Average B-2-B selling price in past 5 years |
23 | Competition from substitute products |
24 | Gross margin and average profitability of suppliers |
25 | New product development in past 12 months |
26 | M&A in past 12 months |
27 | Growth strategy of leading players |
28 | Market share of vendors, 2023 |
29 | Company Profiles |
30 | Unmet needs and opportunity for new suppliers |
31 | Conclusion |
32 | Appendix |